Case Selection
LASSO scenarios focus on specific meteorological regimes, and thus require careful selection of case dates to be simulated. A balance is struck between the number of cases, size of the modeling configuration, and available resources. For LASSO-CACTI, a two-stage process was employed to scan a larger number of case dates with coarser grid spacing followed by targeted simulations at LES scale.
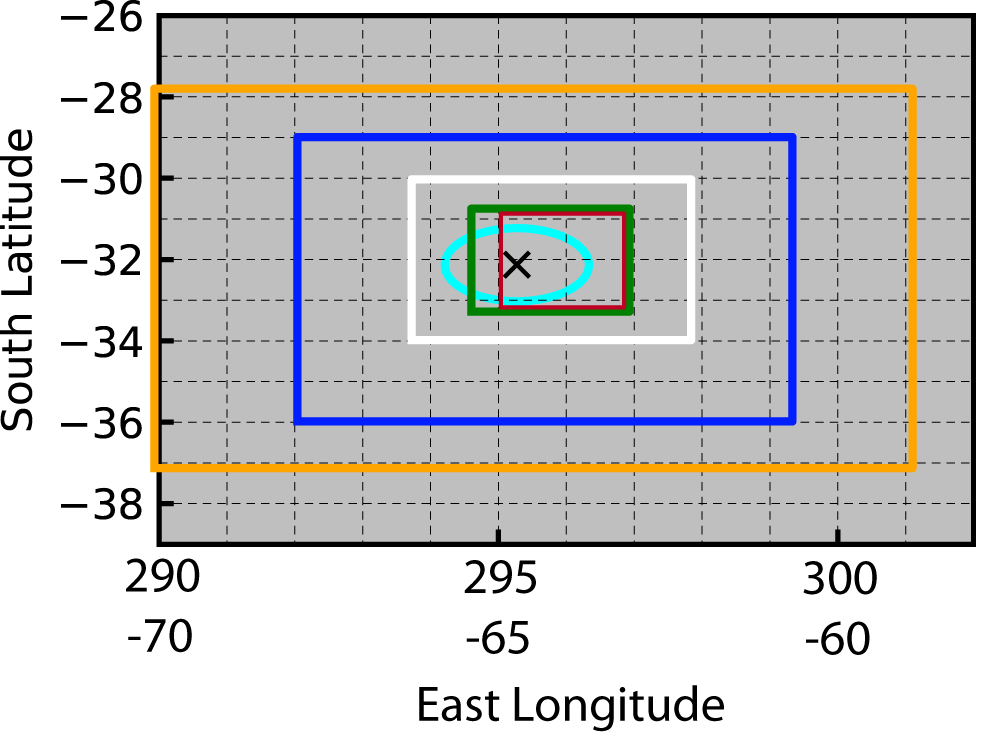
Figure 2 Illustration of WRF nested domains relative to the AMF location (the ‘X’) and ARM C-Band scanning domain (cyan oval). The oval is the projection-distorted ‘circular’ domain for the ARM C Band radar products, which have a ~100-km radius. Note that a ridge blocks the radar signal approximately westward of 295°E (65°W) so that the evaluation region is limited to the red box. The outer colored boxes indicate the four nested WRF domains.
The selection process consisted of the following steps:
A list of candidate dates was obtained from Adam Varble, the CACTI PI. The list considered factors such as convection cases coinciding with data/instrument availability.
Animations of VISST IR satellite data were then inspected for the occurrence of convective initiation and upscale growth occurring within the CSAPR2 observational domain. Cases were not considered when convection only occurred outside of the CSAPR2 domain or when initiation occurred outside of this domain and then advected into it, as the upscale growth would not be observable by ARM instrumentation, and adding value to the ARM measurements is a primary goal of LASSO. The extents of the WRF domains in relation to the region visible to the C-band radar are shown in Figure 2.
A range of convective behavior was sought for which CAPE was used as an index to verify that a sampling of the range had been achieved for the chosen dates. CAPE values (J kg-1) were calculated using both the most unstable layer (MU) and the mixed layer (ML) methodologies. Selections through this stage produced 20 case dates, which we refer to as the “mesoscale cases,” with the list of mesoscale case dates shown in Table 2.
A 33-member ensemble of mesoscale simulations were conducted (∆x=2.5-km grid spacing) for each mesoscale case day. The ensemble members correspond to different large-scale forcings from ERA5 (1 member), FNL (1 member), ERA5-EDA (10 members), and GEFS (21 members) as described in the Initial and Boundary Condition section. Case days with poor ensemble performance were assigned lower priority as the large-scale conditions might not be adequately captured in the available forcings. Some questionable case days were still retained, as it was possible that the LES resolution (∆x=100 m) might improve dynamical features unresolved at 2.5 km that could improve the simulation.
This selection process resulted in nine potentially viable LES case dates, listed in Table 2 as cases that include a letter alongside the case number. These dates have dedicated pages with more extensive descriptions accessed by the links in the table. The descriptions include a snapshot of the satellite IR brightness temperature (at 11.2 μm) aimed at illustrating a distinguishing phase of the development. The remaining cases with only mesoscale ensembles include a short description directly in Table 2.
Note that the black X in Figure 2 indicates the location of the AMF site and the white box indicates the domain used for the Δx=500-m grid (domain 3) in the LES simulations.
Satellite-based animations of brightness temperature for all days are available via the Bundle Browser. Look in the table of simulations for each case date under the “Sim. Tb” column to access animations for the simulations, and look in the “Observation Summary” pages of the case dates for the observed brightness temperature animations.
Case |
Date |
Description |
---|---|---|
1 |
2018-10-26 |
|
2 |
2018-11-04 |
|
3 |
2018-11-05 |
|
4 |
2018-11-06 |
|
5 |
2018-11-10 |
|
6 |
2018-11-21 |
|
2018-11-29 |
|
|
8 |
2018-11-30 |
|
2018-12-04 |
|
|
2018-12-05 |
|
|
2018-12-19 |
|
|
2019-01-22 |
|
|
2019-01-23 |
|
|
2019-01-25 |
|
|
2019-01-29 |
|
|
16 |
2019-01-31 |
|
2019-02-08 |
|
|
18 |
2019-02-23 |
|
19 |
2019-03-14 |
|
20 |
2019-03-15 |
|